Table of Contents
ToggleThe Life Cycle of a Star: From Birth to Supernova and Beyond
Introduction
Stars are the fundamental building blocks of the universe. They are responsible for creating most of the elements found in our world. The life cycle of a star is a fascinating process. From its initial formation in a vast cloud of gas to its end, either as a beautiful nebula or a powerful black hole, each phase tells a unique story. This journey is shaped by one main factor: mass. The mass of a star determines how long it will live, how it evolves, and ultimately how it will end. In this blog, we’ll delve deep into each stage of the life cycle of a star.
The life cycle of a star can vary greatly depending on its initial mass. A star like our Sun will follow a very different path compared to a star much larger than it. Low-mass stars have long, stable lives, while high-mass stars live fast and explode spectacularly. The life cycle of a high mass star involves explosive phases, leading to either neutron stars or black holes. If you want to know more about black holes you can check out our post about understanding black holes This makes studying the life cycle of stars crucial for understanding the broader cosmos.
What Determines the Life Cycle of a Star?
The life cycle of a star is determined primarily by its mass. Mass is the single most important factor that influences every phase of a star’s life. Larger stars have more fuel, but they burn through it quickly, while smaller stars burn slowly and live longer. Let’s explore how mass dictates each stage of a star’s existence.
The mass of a star affects its gravity, temperature, and pressure. These factors determine how a star forms, how long it stays stable, and how it ultimately dies. The greater the mass, the stronger the gravitational pull, which means the core must reach much higher temperatures to balance this pressure. This process of balancing is called hydrostatic equilibrium, and it plays a key role in what determines the life cycle of a star.
High mass stars are usually hotter and more luminous. They shine brightly but exhaust their hydrogen fuel at an accelerated rate. In contrast, low mass stars, like red dwarfs, have a slower fusion process. These stars can shine for billions of years without significant changes. It is this interplay between gravity, fusion, and pressure that determines whether a star ends as a white dwarf, a neutron star, or even a black hole.
Birth of a Star: The Stellar Nursery
Stars begin their lives in enormous clouds of gas and dust, known as nebulae. These regions, sometimes called stellar nurseries, are the birthplaces of stars. The process begins when a disturbance, like a nearby supernova explosion, causes parts of the nebula to collapse under gravity. This collapse leads to the formation of a dense region called a protostar, marking the first stage in the life cycle of a star.
As the protostar continues to collapse, it grows hotter. The increasing temperature eventually reaches a point where nuclear fusion starts. This fusion converts hydrogen atoms into helium, releasing energy in the form of light and heat. At this stage, the star has officially “ignited,” and it begins its life on the main sequence—a stable phase where it spends most of its existence.
This early phase is crucial because it sets the stage for everything that follows. The mass of the protostar, once again, is the deciding factor. If the mass is large enough, the star will continue to evolve through a dramatic series of events. Smaller protostars might never gain enough mass to start fusion, and they become “failed stars,” known as brown dwarfs. The successful formation of a star involves a balance between the inward pull of gravity and the outward push from nuclear fusion, keeping it stable.
Main Sequence Stage: The Longest Phase
After the initial fusion of hydrogen, the star enters what is known as the main sequence stage. This is the longest phase in the life cycle of a star, where it remains stable for millions to billions of years. During this time, hydrogen in the core continues to fuse into helium, producing a tremendous amount of energy. This energy provides the pressure needed to counterbalance gravity, keeping the star from collapsing.
The duration of the main sequence phase depends greatly on the mass of the star. High mass stars, which are significantly hotter and brighter, burn through their hydrogen fuel quickly. As a result, they remain in this phase for a much shorter time compared to low mass stars. For instance, a massive blue giant might stay on the main sequence for only a few million years, while a smaller red dwarf can remain stable for tens of billions of years.
Stars like our Sun are also in the main sequence phase. They are classified as medium-sized stars, and they spend roughly 90% of their lives in this stable stage. Eventually, as hydrogen in the core gets depleted, the star will leave the main sequence and enter a more unstable period. The star’s core will contract, while its outer layers expand, setting the stage for the next phase in its journey.
Stars begin their lives in enormous clouds of gas and dust, known as nebulae. These regions, sometimes called stellar nurseries, are the birthplaces of stars. The process begins when a disturbance, like a nearby supernova explosion, causes parts of the nebula to collapse under gravity. This collapse leads to the formation of a dense region called a protostar, marking the first stage in the life cycle of a star.
As the protostar continues to collapse, it grows hotter. The increasing temperature eventually reaches a point where nuclear fusion starts. This fusion converts hydrogen atoms into helium, releasing energy in the form of light and heat. At this stage, the star has officially “ignited,” and it begins its life on the main sequence—a stable phase where it spends most of its existence.
This early phase is crucial because it sets the stage for everything that follows. The mass of the protostar, once again, is the deciding factor. If the mass is large enough, the star will continue to evolve through a dramatic series of events. Smaller protostars might never gain enough mass to start fusion, and they become “failed stars,” known as brown dwarfs. The successful formation of a star involves a balance between the inward pull of gravity and the outward push from nuclear fusion, keeping it stable.
Life Cycle of a Low Mass Star
Once a star depletes the hydrogen in its core, it moves on to the next phases of its life. For a low mass star, like our Sun, the transition is relatively slow and steady. These stars have a peaceful end compared to their massive counterparts. When hydrogen is exhausted, the core contracts while the outer layers expand, and the star becomes a red giant.
In the red giant phase, helium fusion begins. The core contracts even further, generating enough heat to fuse helium into carbon and oxygen. This phase is characterized by a dramatic expansion of the star’s outer layers, which become cooler, giving the star a reddish hue. The red giant can grow large enough to engulf any planets that are in close orbit, including, hypothetically, Earth when our Sun reaches this stage.
Eventually, helium fusion in the core also runs out, and the star sheds its outer layers, forming a beautiful shell of ionized gas known as a planetary nebula. What remains is the dense, hot core—now called a white dwarf. This white dwarf will gradually cool down over billions of years, fading slowly until it becomes a cold, dark remnant known as a black dwarf.
Life Cycle of a High Mass Star
The life cycle of a high mass star is significantly different from that of a low mass star. High mass stars have much shorter lives, but their deaths are far more spectacular. After spending a brief time in the main sequence phase, high mass stars rapidly exhaust their hydrogen and move on to fuse heavier elements.
Once hydrogen runs out, the star becomes a red supergiant. The core contracts, and temperatures rise enough for helium to begin fusing into heavier elements like carbon and oxygen. This process continues in multiple cycles, fusing elements as heavy as iron in the core. Each fusion stage is shorter than the last, with the final stage—iron fusion—leading to instability. Iron cannot be fused to produce energy, which leads to the collapse of the star’s core.
The core collapses suddenly, triggering an immense explosion known as a supernova. This explosion is one of the most energetic events in the universe, releasing incredible amounts of light and energy. The outer layers are blasted into space, and the core is left behind. Depending on the remaining mass, this core could become either a neutron star or a black hole. A neutron star is incredibly dense, composed mostly of neutrons, while a black hole is a region where gravity is so strong that nothing—not even light—can escape.
The End of a Star: White Dwarfs, Neutron Stars, and Black Holes
As a star reaches the end of its life, its fate depends largely on its mass. Different masses lead to different types of stellar remnants. Let’s take a closer look at what happens during the final stages in the life cycle of a star.
For low mass stars, like our Sun, the end is relatively quiet. After the red giant phase, the star sheds its outer layers, which drift away into space, forming a beautiful planetary nebula. What remains is the hot, dense core, now called a white dwarf. White dwarfs are about the size of Earth but incredibly dense, with no nuclear fusion taking place. Over time, they cool down and eventually become black dwarfs, although this process takes billions of years.
For high mass stars, the end is far more dramatic. After the supernova explosion, the core that remains can either become a neutron star or collapse further into a black hole. Neutron stars are incredibly compact, with a radius of just a few kilometers but masses greater than that of our Sun. They spin rapidly and often emit beams of radiation, creating pulsars. On the other hand, if the core’s mass is great enough, gravity causes it to collapse into a singularity—a black hole—from which not even light can escape.
These stellar remnants play crucial roles in the cosmos. White dwarfs provide insight into the later stages of stellar evolution, while neutron stars and black holes are key subjects in the study of extreme gravitational phenomena. The formation of black holes and neutron stars also contributes to the cosmic environment, enriching the galaxy with elements produced during the star’s life and violent death.
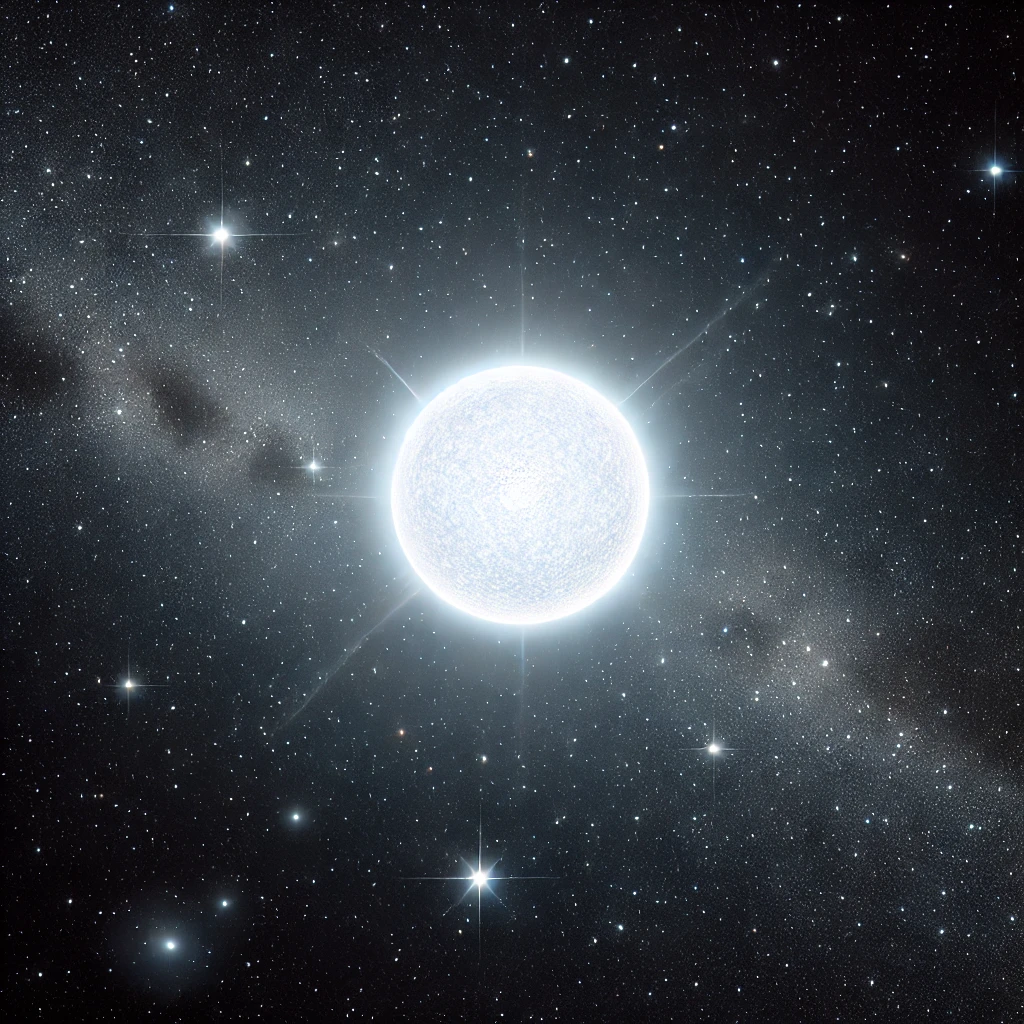
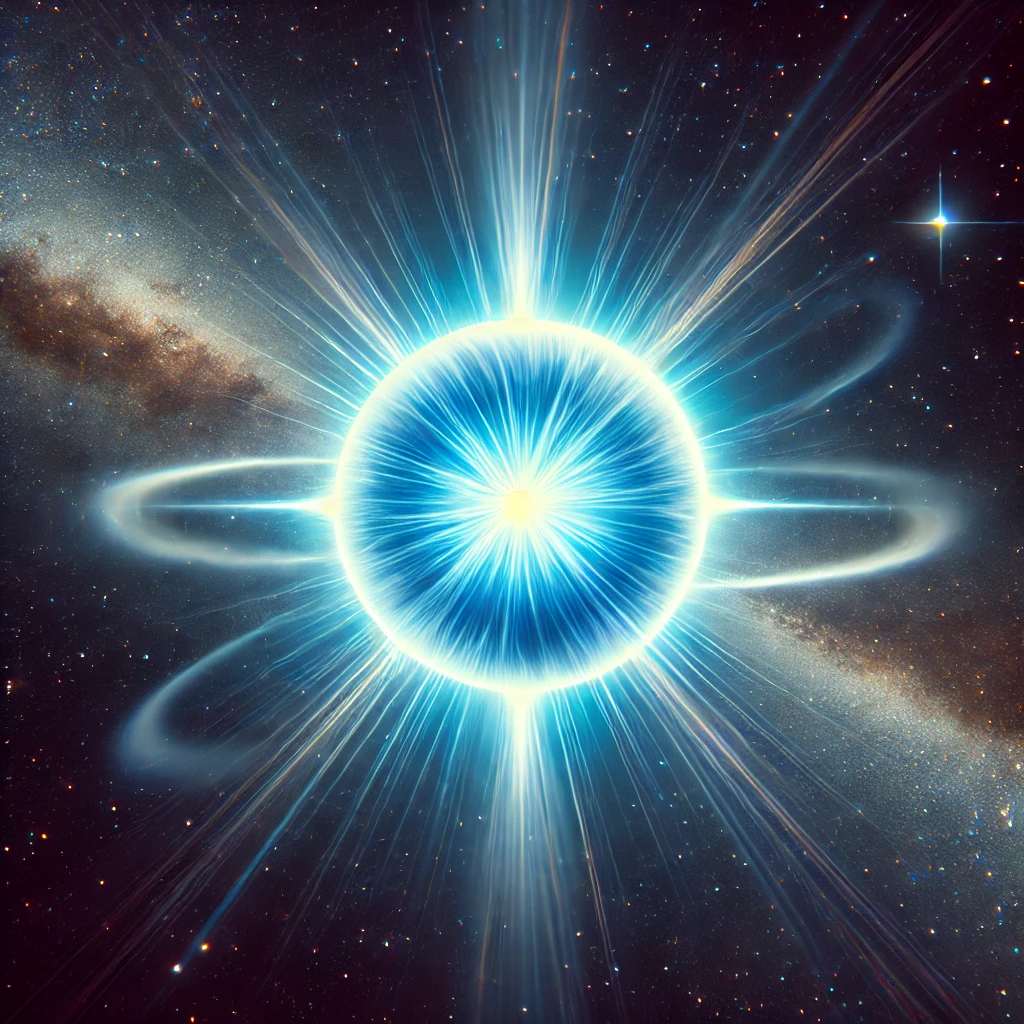
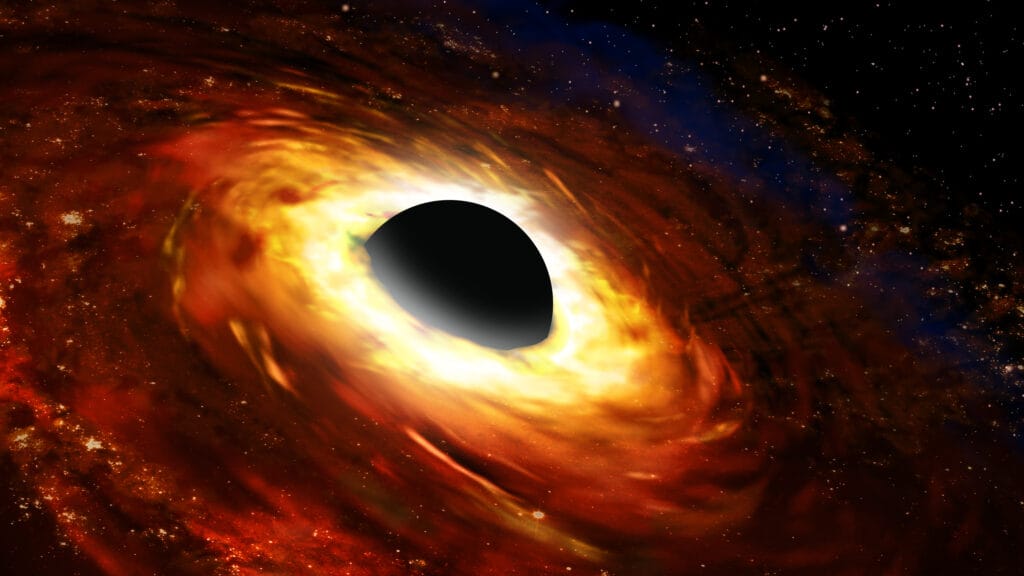
Stellar Remnants and Their Impact on the Universe
The life cycle of a star doesn’t end quietly; even after a star’s death, it leaves a profound impact on the universe. The remnants of stars—whether they are white dwarfs, neutron stars, or black holes—continue to shape the cosmic landscape. These remnants play crucial roles in the formation of new stars, planets, and even the complex chemistry that leads to life.
One of the most important contributions of stellar remnants comes from supernova explosions. When high mass stars explode in a supernova, they scatter elements such as carbon, oxygen, iron, and other heavy elements into space. These elements become part of interstellar clouds, which are the birthplaces of new stars and planetary systems. Without these heavier elements, planets like Earth and, ultimately, life as we know it, would not exist. Thus, the life cycle of a high mass star contributes directly to the materials necessary for creating the next generation of stars and planets.
White dwarfs, on the other hand, serve as cosmic clocks. By observing and studying white dwarfs, astronomers can estimate the age of star clusters and even the galaxy. Neutron stars also have unique roles—they are responsible for the creation of extremely dense environments where exotic phenomena, like pulsars, can be observed. Pulsars are neutron stars that emit beams of radiation, and their regular emissions make them precise cosmic lighthouses, valuable for studying the universe’s structure.
Black holes, the most mysterious of stellar remnants, continue to intrigue scientists. They affect the regions around them with their immense gravitational pull, sometimes pulling in nearby gas and dust to form an accretion disk. This process generates enormous amounts of energy, and black holes are believed to be the engines powering quasars—the brightest objects in the universe.
Life Cycle of Star vs. Life Cycle of a High Mass Star
The life cycle of a star can vary significantly based on its initial mass. Stars come in a wide range of masses, and this difference leads to distinct life paths. Let’s compare the life cycle of an average star, like our Sun, to the life cycle of a high mass star, and understand the key differences in their evolution and end states.
A typical low mass star begins its life in a nebula and forms a protostar that eventually stabilizes on the main sequence. Low mass stars, such as red dwarfs and stars similar to our Sun, spend billions of years in this main sequence phase, fusing hydrogen into helium at a steady rate. Once hydrogen is depleted, they expand into red giants. Eventually, they shed their outer layers, leaving behind a white dwarf. This process is relatively calm compared to that of a high mass star. Low mass stars end their lives quietly, without much drama, as white dwarfs that slowly fade away over billions of years.
In contrast, the life cycle of a high mass star is much more explosive and shorter in duration. High mass stars have more fuel, but they burn through it very quickly, resulting in a relatively brief time in the main sequence phase. After hydrogen depletion, they expand into red supergiants and begin to fuse heavier elements in a series of rapid cycles. This process continues until iron forms in the core. Iron fusion does not release energy, leading to core collapse and an ensuing supernova explosion. The remnants of a high mass star can become a neutron star or a black hole, both of which are far more extreme than a white dwarf.
The key difference lies in how the mass affects both the duration and the nature of each evolutionary phase. High mass stars evolve and end in a spectacular manner, affecting their surroundings dramatically. Low mass stars, however, evolve slowly and end without violent events. Both types of stars contribute to the evolution of the universe, but their contributions are distinct. While high mass stars create the heavier elements through supernova explosions, low mass stars provide stability and contribute lighter elements to the interstellar medium.
The Life Cycle of Stars and the Evolution of Galaxies
The life cycle of a star not only shapes the fate of individual stars but also plays a vital role in the evolution of entire galaxies. Stars are the building blocks of galaxies, and their formation, life, and death create a dynamic environment that influences galactic development. Understanding the different stages in the life cycle of stars helps us comprehend the larger processes that shape the universe as a whole.
During the birth of stars in nebulae, large amounts of gas and dust are drawn together by gravitational forces. These stellar nurseries are often found in the spiral arms of galaxies, where new stars are constantly being formed. The presence of new stars illuminates these regions, creating beautiful structures like the Orion Nebula. As stars mature and enter their main sequence phase, they contribute to the overall brightness of the galaxy. The life cycle of star clusters, composed of hundreds or thousands of stars, also affects the distribution of mass and energy within a galaxy.
When stars die, particularly massive ones that go supernova, they release immense amounts of energy and material. These supernova explosions distribute heavy elements throughout the galaxy. The materials ejected from dying stars enrich the interstellar medium, providing the building blocks for the next generation of stars and planets. This recycling process is crucial to galactic evolution, as it increases the diversity of elements available for star and planet formation. For instance, elements like carbon, oxygen, and iron, which are essential for life, are all produced in the cores of stars and released into space during these explosive events.
Black holes, which are sometimes the remnants of massive stars, can also significantly influence galaxy evolution. Many galaxies, including our own Milky Way, have a supermassive black hole at their center. These black holes can affect the movement of stars, as well as the distribution of gas and dust within the galaxy. The life cycle of a high mass star, leading to a black hole, can thus have a profound effect on the galactic structure, creating an interplay between gravity and energy distribution that shapes the galaxy over billions of years.
FAQs Section
The primary factor that determines the life cycle of a star is its mass. The mass of a star at the time of its formation dictates how it will evolve, how long it will live, and the nature of its eventual end. High mass stars tend to have short but dramatic lives, while low mass stars live longer and end more quietly.
The duration of each stage in the life cycle of a star depends largely on its mass. A low mass star, such as a red dwarf, can spend tens of billions of years in the main sequence phase, while a high mass star, like a blue giant, may only spend a few million years before exhausting its hydrogen. The red giant phase for low mass stars can last for a few hundred million years, while the more intense supergiant stage for high mass stars is much shorter.
The life cycle of a high mass star differs significantly from that of a low mass star. High mass stars burn through their fuel quickly, leading to a brief, explosive life that ends in a supernova. The remnants can become neutron stars or black holes. Low mass stars, on the other hand, burn fuel more slowly, evolve into red giants, and end as white dwarfs. The contrast lies in both the duration of their phases and the dramatic nature of their deaths.
After a star becomes a red giant, it will either shed its outer layers to form a planetary nebula, leaving behind a white dwarf, or, in the case of a high mass star, continue fusing heavier elements until it eventually goes supernova. The specific path depends on the mass of the star. Low mass stars evolve into white dwarfs, while high mass stars face a more violent end.